The KATRIN (Karlsruhe Tritium Neutrino Experiment) team has published the most accurate measurement to date of the upper limit of the neutrino mass in the journal Science, establishing it at 0.45 electronvolts (eV), less than a millionth of the mass of an electron. The KATRIN experiment, launched in 2018 in Germany, will finalise its neutrino mass measurement campaign this year, having reached 1,000 days of data acquisition.
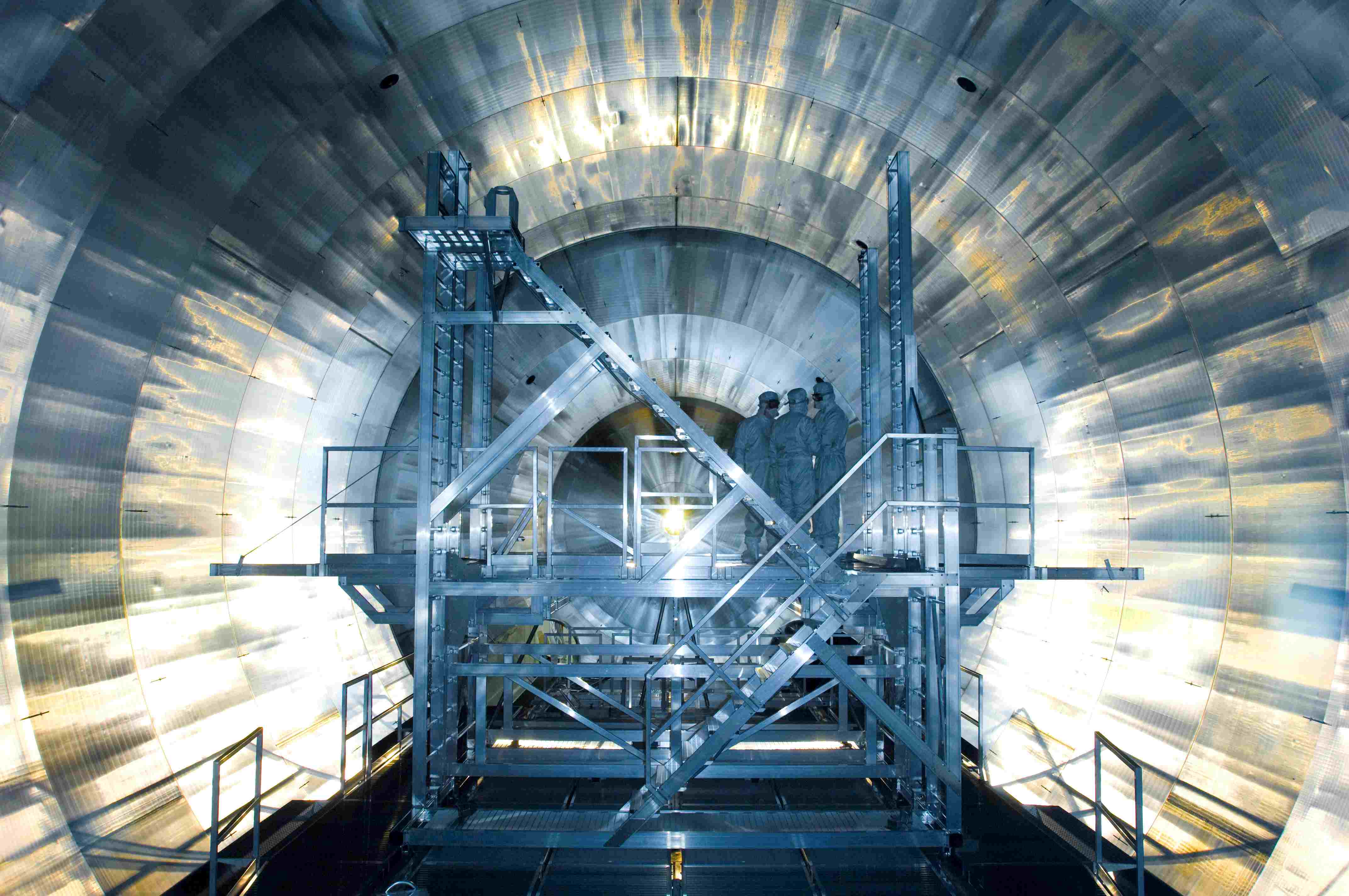
Juan José Hernández Rey - KATRIN neutrinos EN
Juan José Hernández Rey
Research professor at the Spanish National Research Council (CSIC) at the Institute of Corpuscular Physics (IFIC) in Valencia (University of Valencia-CSIC), co-principal investigator of the KM3NeT research team at IFIC and co-leader of the VEGA Group at IFIC
The new result of the KATRIN collaboration further limits the mass of neutrinos. It is a very solid result, both because of the careful analysis of the experimental data and because of the method used, namely the analysis of the energy spectrum in the disintegration of tritium, which requires very few auxiliary hypotheses. It is likely that KATRIN will approach 0.3 eV sensitivity by the end of 2025 but, unfortunately, that will be the limit of its reach.
The significance of the result may seem relative, as the combined data from different cosmological experiments seem to indicate that the sum of the neutrino masses has to be at least almost ten times lower (0.05 eV), but the method used by KATRIN is much more direct and ‘less model dependent’, in the jargon of physicists.
Knowing the exact mass of neutrinos will give us new clues as to what theory lies beyond the Standard Model of Particle Physics. Thus, for example, a hypothesis known as the seesaw mechanism directly links their minuscule mass with very high-energy physical phenomena, which makes neutrinos a gateway to the long-awaited ‘New Physics’.
Raúl Jiménez - KATRIN neutrinos EN
Raúl Jiménez
ICREA research professor of Cosmology and Theoretical Physics at the Institute of Cosmos Sciences at the University of Barcelona, visiting professor in the Astrophysics group at Imperial College London (United Kingdom), and researcher at the Imperial Centre for Inference and Cosmology (ICIC)
The KATRIN underground experiment reports a new upper limit on the mass of the electron neutrino (in nature there are three families of neutrinos: electron, muon and tau; the Katrin experiment is only sensitive to measuring the mass of the former) of 0.45eV. For the three families of neutrinos the total limit is < 1.35eV. This is an interesting result as it brings the limit for the total mass of neutrinos measured in the laboratory closer to the results in cosmology. In cosmology we have been measuring the total mass of the three families of neutrinos for decades using their effect on the growth of the structures of the universe. This is a different and complementary method to that of KATRIN. Recently, the DESI experiment has reported limits on the total mass of the three neutrinos of < 0.064 eV, which is a factor of 21 smaller than the limit measured by KATRIN. Even so, the KATRIN experiment explores a completely different avenue to cosmology and that is why its result is so relevant, as it brings the limit measured in the laboratory closer to that of cosmology. If one is more conservative in cosmology and wants to relax their assumptions, then the upper limit is < 0.16 eV which is still an 8-fold smaller factor than that of KATRIN.
What this tells us is that, for the moment, there are no surprises: the KATRIN limits are compatible with the most restrictive limits of cosmology and, therefore, there is no room for exotic physics that would imply that neutrinos in the cosmos behave differently than on Earth. Unfortunately, KATRIN in its final measurement will only reach the limit of < 0.9 eV and will remain far from the cosmological limits. However, there are other experiments on Earth that may very soon bring us closer to a better understanding of the nature of neutrinos and their masses. These are the NEXT experiment, which is located in the Spanish-built Canfranc Underground Laboratory, and the HyperKamionkande experiment in Japan, where the Canfranc Underground Laboratory plays a crucial role.
Underground experiments in conjunction with cosmology will bring us closer to discovering the nature of neutrinos over the next five years and thus understanding nature itself, and why there is matter in the universe and not just radiation. It is in this context that the relevance of KATRIN's results must be framed.
David Alonso González - KATRIN neutrinos EN
David Alonso González
Predoctoral researcher at the UAM-CSIC Institute of Theoretical Physics and in the Department of Theoretical Physics at the Autonomous University of Madrid
The neutrino is an extremely light elementary particle with no electrical charge that interacts very weakly with ordinary matter, which makes it extremely difficult to detect. Despite this, it plays a crucial role in astrophysical and cosmological phenomena and its study offers a lot of information about physics beyond the Standard Model, the most complete experimentally confirmed theory of particle physics that we currently have. The theoretical prediction that neutrinos could oscillate between different types or ‘flavours’ (electronic, muonic or tauonic neutrino) — a phenomenon that could only be explained if they had mass — was confirmed experimentally by the Super-Kamiokande and SNO collaborations, which were recognised with the Nobel Prize in Physics in 2015 for Takaaki Kajita and Arthur B. McDonald.
Although neutrino oscillation experiments allow the differences between the squared masses of the different neutrino flavours to be measured, they do not allow a direct study of the absolute scale of their mass. In this sense, only the combination of various cosmological observations allows an upper limit to be set on the sum of the masses of all neutrinos within the framework of standard cosmology. Motivated by this limitation, since the 1990s, attempts have also been made to directly measure the neutrino mass through experiments on Earth that study the disintegration of atomic nuclei, especially tritium. This is the case of the pioneering experiments in Mainz, Troitsk and, more recently, KATRIN.
In this article, KATRIN presents its latest results in the measurement of the effective mass of the electron antineutrino (the antiparticle of the electron neutrino, which will have the same mass) through the integral reconstruction of the beta decay of tritium, one of the lowest energy decays.
In this decay, a neutron transforms into a proton, emitting an electron and an antineutrino whose combined energy is 18.6 keV (kiloelectronvolts). By measuring the electron's energy with great precision, KATRIN infers, thanks to the principle of conservation, the amount of energy carried by the antineutrino. The mass of the neutrinos would modify the energy distribution of these particles. Specifically, the maximum energy that electrons can reach (the so-called tail of the decay spectrum) would be slightly reduced with respect to what they would reach if neutrinos had no mass. This effect is extremely subtle and, in fact, has not been directly observed, which prevents obtaining an exact measurement of the mass of neutrinos and only allows upper limits to be established.
After 259 days of measurements of more than 36 million electrons, KATRIN combines the results of five data collection periods to establish an upper limit on neutrino mass of 0.45 eV (less than one millionth of the mass of the electron), reducing its own previously published limit by a factor of 2. The collaboration aims to improve this result even further by completing the planned 1,000 days of data collection. The article explains in detail the technical characteristics of the experiment, as well as the meticulous handling of statistical and systematic uncertainties in order to derive the limit.
In conclusion, although it does not introduce radically new ideas with respect to previous publications, KATRIN refines the precision of the method and, by increasing the measurement time, manages to improve its results and continues on the path to determining a fundamental parameter in particle physics such as the mass of neutrinos.
- Research article
- Peer reviewed
KATRIN Collaboration.
- Research article
- Peer reviewed